The Sustenance of Space
An Exploration of NASA’s Plant Programs
Part 3
Editor’s Note
Space may be the final frontier, but we rarely imagine it associated with dining—or perhaps with eating at all. Of course, it is one of the many considerations NASA researchers have on their minds, thankfully for those who need to be fed on their missions. The Life & Thyme editorial team was welcomed to the Kennedy Space Center where researchers are exploring exactly that. Today is the final installment in a three-part series about the organization’s efforts to that end, as well as growing food on the way to and on the surface of Mars.
Words by GIA HUGHES
Photography by ANTONIO DIAZ
Videography by AUSTIN STRAUB
Exploring the facilities inside the Space Station Processing Facility left us wondering about the eventual destination in plant sustenance in space: Mars. How will the scientists who eventually inhabit the planet incorporate the water systems studied by Veggie? How will the roots of a plant develop, as studied in APEX-06? Or how can nutrient-infused Arcillite be used in place of soil? All experiments must work in conjunction to assist in successfully growing and sustaining life on a new planet.
During our visit to the Kennedy Space Center we learned plants will need an artificial Earth-like atmosphere and environment in order to grow and thrive in space. NASA is studying just how much carbon dioxide, light, nutrients and water—to name a few essential parts—a plant will need in order to sprout and grow to maturation, from seed to dinner plate.
LONG DURATION FOOD PRODUCTION LAB
The last stop during our visit to Kennedy Space Center is the Long Duration Food Production Lab. As we walk through the halls, each passing room subtly marked with a small sign, we make our way to a brightly lit white-walled room, met by Ralph Fritsche, Project Manager for Food Production. “This is where we’re going to,” he greets us, referring to Mars, giving us context into where his studies fit into plant growth in space.
We are in the room in which research is done to study food growth and production during a long-duration spaceflight. “That’s both the pathway to Mars, and then ultimately the destination at Mars,” Fritsche explains.
A large shelved structure exists in the corner, serving as an experimental system for testing various setups and methods for water delivery. One consistent challenge scientists face while studying plant growth in space is getting air and water to the root zone of the plants grown in man-made ecosystems. Through the use of controls, NASA will be able to compare various methods of successfully and sustainably irrigating plants on a long-duration flight, as well as on the surface of a foreign planet. And once they confirm the most viable solution, they’ll be able to select the best plants to prepare for space flight. It’s important to note, however, the plants grown in space flight will not replace the diet of the astronauts, but will provide supplemental nutrition for them.
“The pre-packaged food the folks at [Johnson Space Center] prepare for the crew will meet the bulk of the caloric requirements,” says Fritsche. “What we’re going to be doing is trying to provide key nutrients for periods of long-duration spaceflight—three years or more.” Over the course of these years, these essential nutrients become less viable; the food’s nutrients begin to degrade after such an extended period of time. “What we’re going to do is target some of those key nutrients with plants we’ve demonstrated can grow in the environment of deep space,” Fritsche continues.
Up until now, NASA has grown plants in the low earth environment of space. But there are new challenges the farther you get from Earth. “You start worrying about the radiation impacts of deep space and things the Van Allen belt protect us from,” Fritsche says.
Once you are on the surface of Mars, this issue with water delivery in microgravity dissipates. “The water and air behave more traditionally like they do on Earth,” Fritsche explains.
Growing plants on Mars will closely resemble how plants are grown here in the Long Duration Food Production Lab. A hydroponic system for watering—much like what is used for larger food farming production systems on Earth—will be tested, for example. The scientists will compare the Earthbound hydroponic systems tested in the lab to what it would take if you combined it with Martian regolith—which is void of organics—and converted it into a usable soil through the incorporation of nutrients and fertilizers.
The Long Duration Food Production Lab has “a wealth of data from the rovers and the orbiting systems on Mars. From that data we can actually develop simulants that are more accurate from an agricultural perspective,” explains Fritsche. They need to learn how to develop these simulants, how to remove any negative factors from the regolith, and how to augment it with minerals and nutrients essential to plant growth here on earth.
“Once you start staying there for a longer period of time, it gets just too costly to bring the food there,” Fritsche says. When you’re trying to sustain these crewmembers over an extended period of time, those costs can be “fairly prohibitive,” according to Fritsche. Logistically, fiscally and sustainably, it would make the most sense to grow food on the surface of Mars.
The pick-and-eat crops NASA will be focusing on for potential Martian sustenance include leafy greens, microgreens (which they are growing in the laboratory in front of us; they’re able to condense more nutrients into a smaller area with plants like these), and crops like radishes, potatoes, tomatoes and peppers. Crops are researched in partnership with Fairchild Tropical Botanical Gardens. “They just tested dozens of crops in schools around Florida and we distilled through their data to come up with possible ones to include,” says Matt Romine, plant physiologist and biologist. “For instance, we have garden amaranth. It’s very high in lutein and zeaxanthin. These are important things for preventing macular degeneration in orbit.”
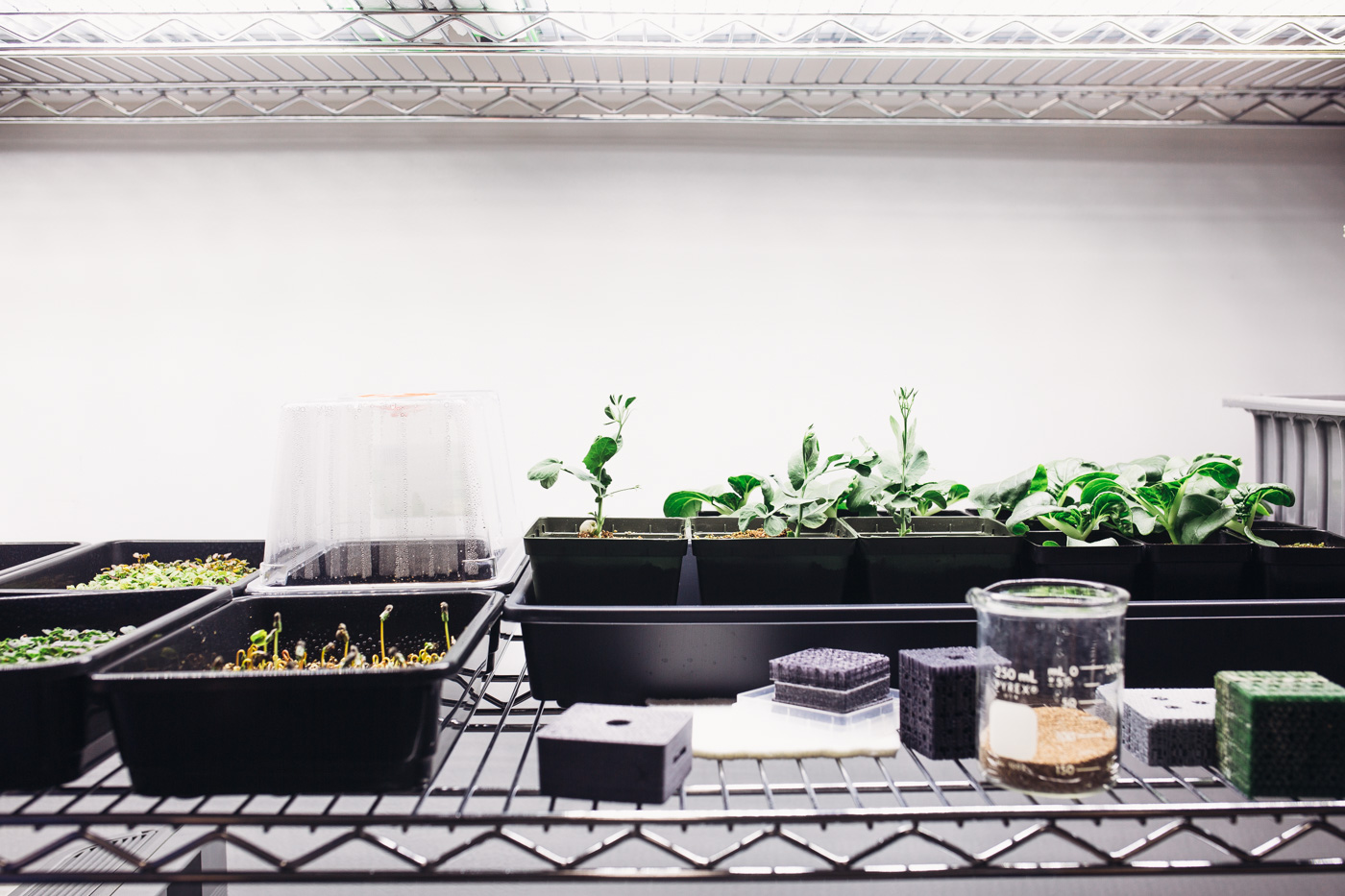
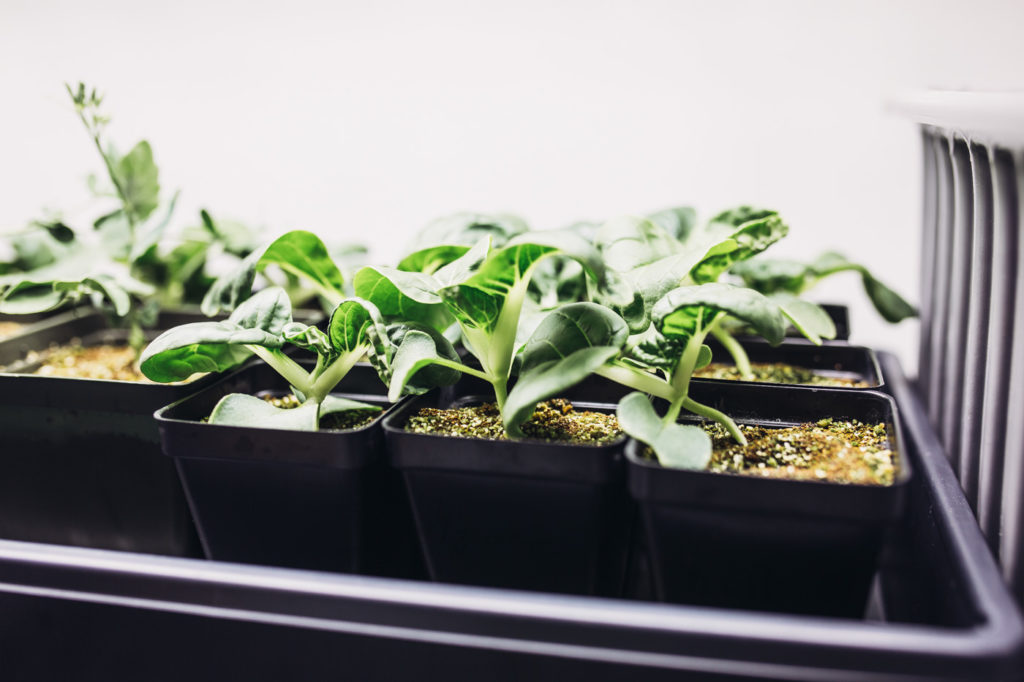
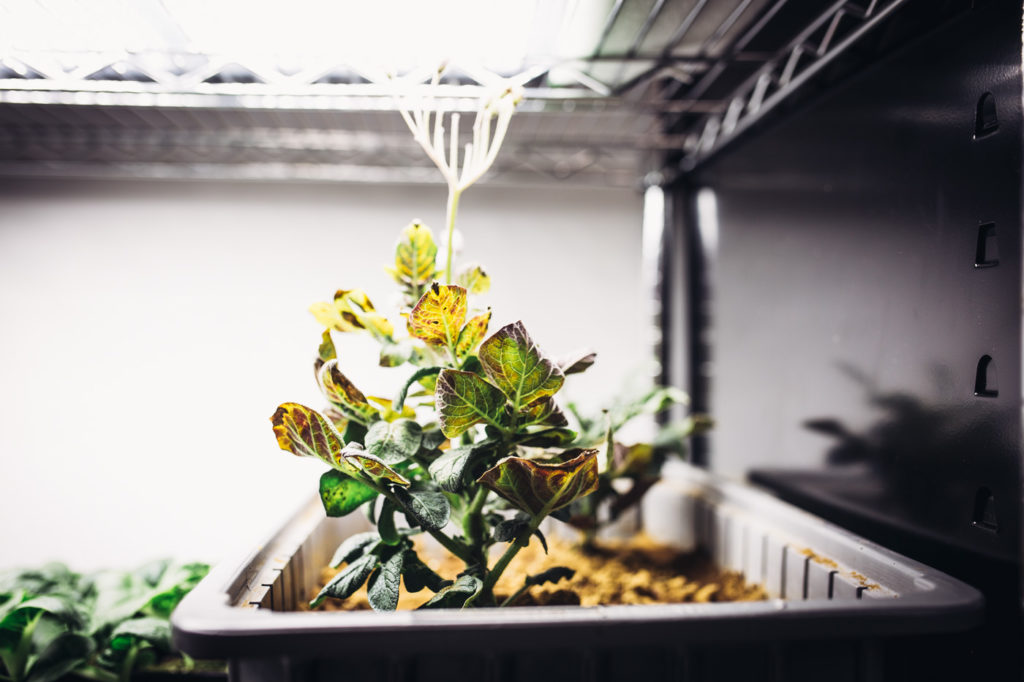
The first time we land on Mars will likely be a brief stay. Much of the food will be brought along on the long-duration space flight. “After we start staying on the surface for longer durations of time,” Romine explains, “we start actually having dedicated spaces and modules for food production activities.” The plants potentially could be grown in a greenhouse-like structure. “It might be underground in something like a lava tube; that’s been proposed as a way to avoid the radiation effects,” says Romine. They could also potentially be grown in an “aluminum cell attached to the side of the habitat with a lot of LED lights in it.” The exterior of the greenhouse would not be clear, however. There is so little sunlight on Mars that the lighting will have to be entirely artificial.
“There’s also some technologies here we’re experimenting with,” says Fritsche. One is in reference to handling seeds in microgravity. You can’t simply pack jars or packages of seeds. What would happen when you opened them?
Seeds can be embedded into a polymer called Polunin—a sticky substance like tape. “You can make these with any seeds you choose and whatever number of seeds you want,” says Fritsche. “And within the Polunin polymer are some nutrients that will help them start off, as well as augment what’s already in the seeds.” The eventual goal is to be able to grow seeds to maturation, and then reuse their seeds to grow new plants.
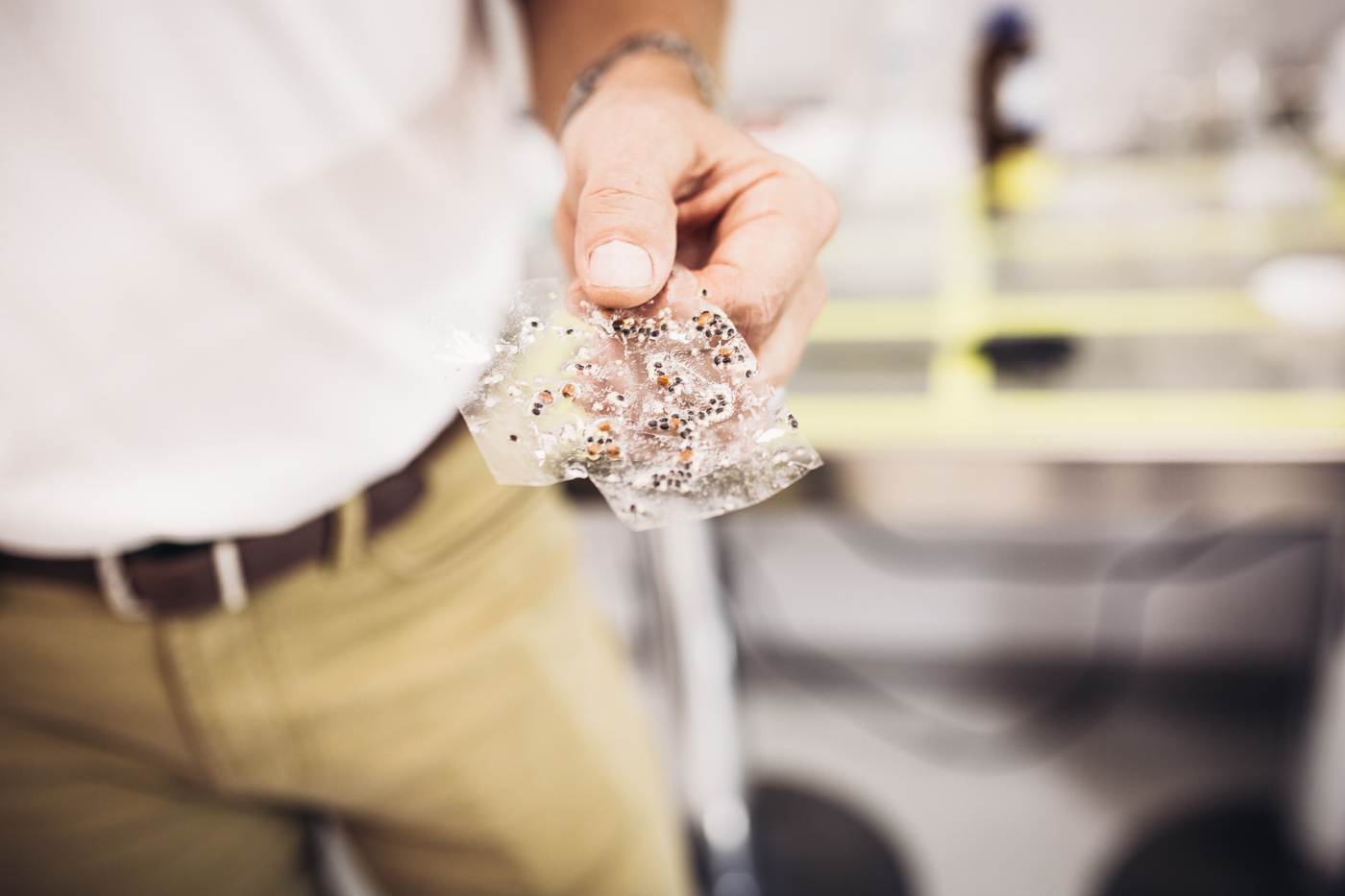
“We like to collaborate with a lot of universities,” says Fritsche. One subject these partnerships have explored is the question of water and air delivery. For example, Utah State created a 3D-printed substrate with geometry that allows water to wick up. “The water stays in the triangle vertices, but the central area of the triangle is open to air,” says Fritsche. “This is one way to passively get air and water to the root zone in such a way that the plants get what they need.”
Fritsche continues, “We’re going to still be looking at an active solution where we actually have plants telling us with sensors when they need water, and then the equipment will provide the water to them.” But for smaller plants like microgreens, a passive solution is ideal because it does not require any power. “If we can figure out how to make the appropriate shapes, geometry, and what seeds would work, potentially you could passively provide them with the air and water they need,” Fritsche continues.
There is a 3D printer on orbit, so files could be sent and materials could be printed during space-flight. “The same company that has the 3D printer is planning on flying a recycler, so once you’ve grown things with that material you could potentially recycle it, print it again, and grow it again,” says Fritsche. They are also currently looking into studying running cellulose acetate through a 3D printer. “Cellulose acetate would basically be something we would derive from the non-edible biomass that was a result of the plants we grew,” says Fritsche. You could take the non-eaten plants, process them, and create a medium to grow your plants on. “Now you’re getting to become more Earth independent,” says Fritsche.
Fritsche then gestures to a machine across the room: an automated farming machine known as FarmBot. The scientists at Kennedy Space Center often look to the Earthbound commercial world—such as hydroponic farming, for example—and incorporate certain systems in space. “We have a paradigm in our minds of spaceflight driving all the technology that goes back into the commercial world,” says Fritsche. “But nowadays, because of the way things are, how technology is open-sourced, and how there’s a lot of innovation that takes place, it works both ways.”
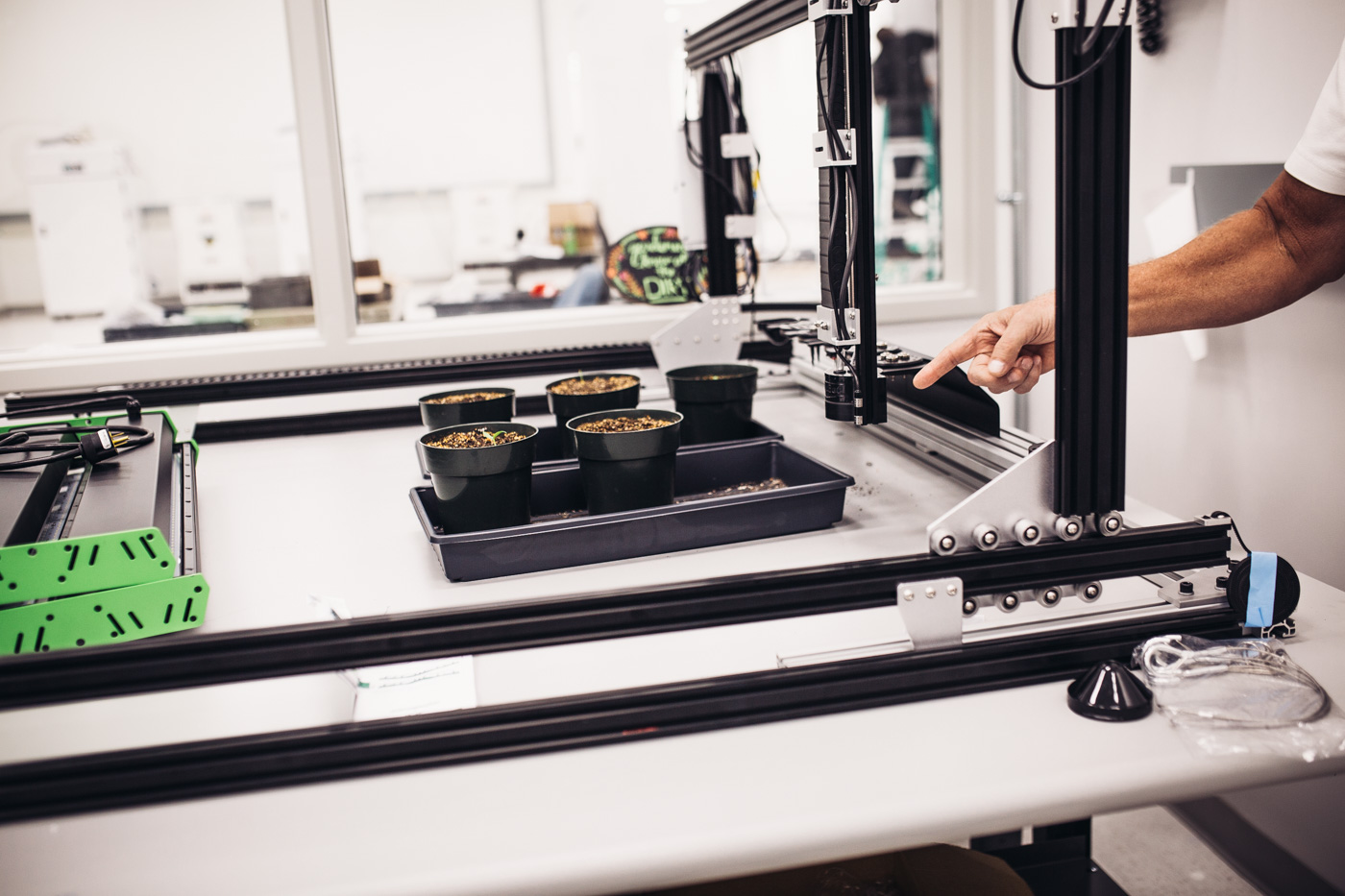
Developed by a small company out of California, FarmBot automizes the growing of crops. “There’s a software interface where you can select whatever crops you want to grow. Like a videogame, there’s the grid of the growing area and you can lay out the crops where you want on the software,” says Fritsche. “Part of the software is a regimen for what any one of those crops needs for its full cycle, from the point it was planted to the point it’s harvested.” If you had one of these in space and you had a source for electricity, you could automate crops—something Fritsche constantly wonders the possibility of.
“I want the crew to have some involvement in growing their food because there’s a lot of psychosocial benefits for them being involved in activities that are reminiscent from Earth,” Fritsche continues. “But you don’t want them to be consumed by essentially farming continuously.” So they’re looking as what aspects of automation can be applicable to food production on Mars. This machine shows how developments on this planet can be used on the next. It could be a sign of humanity—and a source for humanity—on Mars.
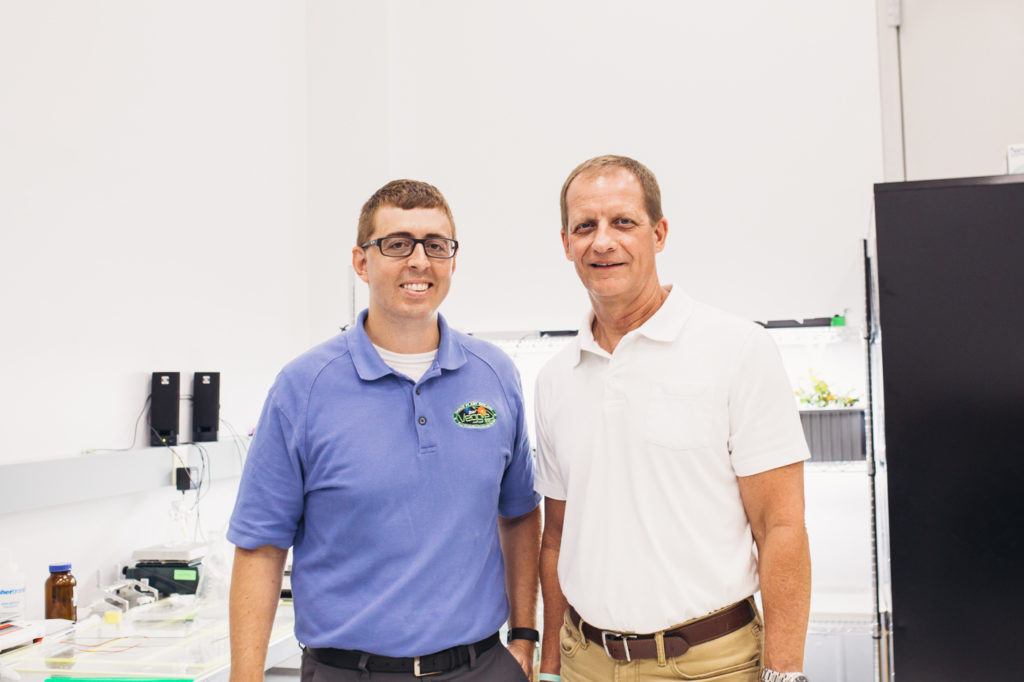
__________
As we leave the Space Station Processing Facility, we have to wait out a weather warning. We bolt to the van we arrived in and quickly throw our camera gear into the trunk, our clothes and hair soaked through. Minutes later, the storm passes and all is calm again other than the remaining sign of water bustling down the swampy irrigation channels near the road shrouded in vibrant greenery.
We leave NASA that day reeling with facts and numbers and hypotheses. We sit in silence for some of the drive back to our hotel, processing everything we had spent the day learning. Above all else, we learned to never lose the hope of wonder. To constantly question what can be possible. And to try until you find a way. And in this case, a way to bring life to Mars. Caring for life sustains life—whether in a lab on Earth, on a space station, or on a planet 140 million miles away.
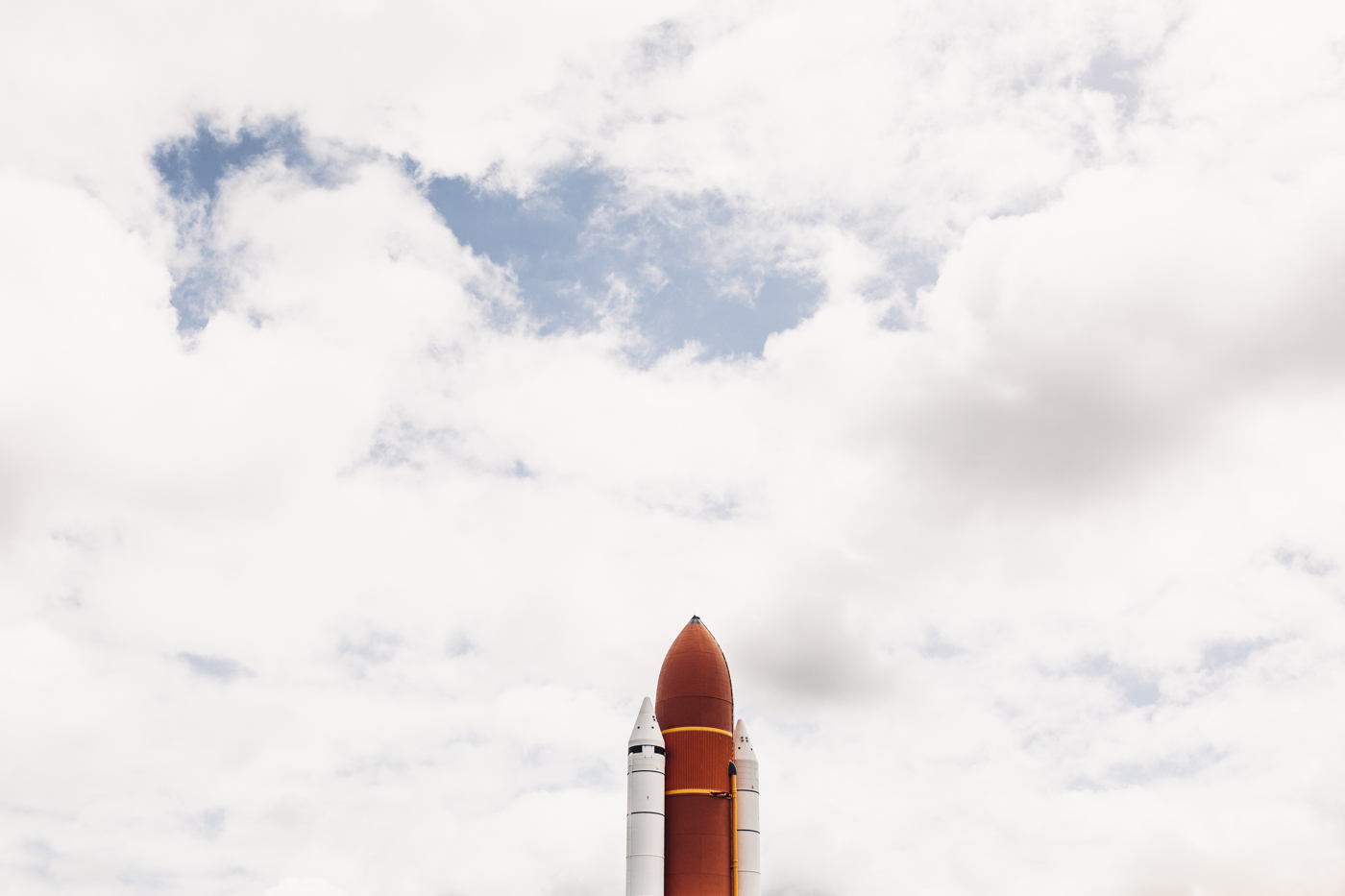
Our comments section is for members only.
Join today to gain exclusive access.